The Expanding Debris Cloud From the Kilonova Tells the Story of What Happens When Neutron Stars Collide
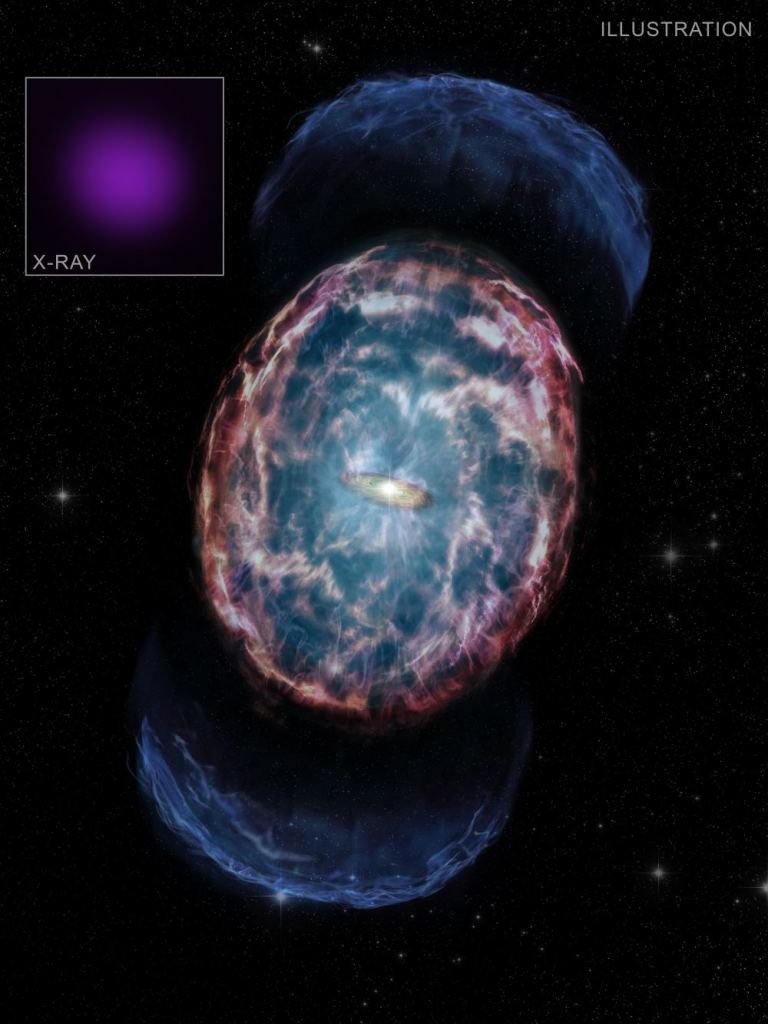
When two neutron stars collide, it creates a kilonova. The event causes both gravitational waves and emissions of electromagnetic energy. In 2017 the LIGO-Virgo gravitational-wave observatories detected a merger of two neutron stars about 130 million light-years away in the galaxy NGC 4993. The merger is called GW170817, and it remains the only cosmic event observed in both gravitational waves and electromagnetic radiation. Astronomers have watched the expanding debris cloud from the kilonova for years. A clearer picture of what happens in the aftermath is emerging. A team of researchers have pieced together the story of GW170917 in a paper titled “The emergence of a new source of X-rays from the binary neutron star merger GW170817.” The lead author is Aprajita Hajela, a graduate student in the Department of Physics & Astronomy at Northwestern University. The paper is published in The Astrophysical Journal Letters. Over the years, astronomers have trained a whole suite of scientific eyes on the expanding cloud, uncovering more and more detail about these cosmic calamities. GW170817 is an unprecedented opportunity to study the kilonova phenomena because astronomers observed gravitational waves and electromagnetic radiation from the merger. The gravitational waves (GW) tell researchers about pre-merger activity, and the electromagnetic observations tell them about the post-merger physical properties. When two neutron stars merge, it produces a cloud of debris and a burst of light called a kilonova. A pair of astronomers introduced the model for neutron star mergers in 1998. They said that the mergers synthesized radioactive nuclei that provide a long-term heat source for the expanding debris envelope. The optical and infrared light in the kilonova comes from the decay of elements like platinum and gold created during the merger. When LIGO and Virgo detected GWs from GW170817, other telescopes detected the optical and infrared light hours later. This is the first optical image ever to show an event initially detected as a Gravitational Wave (GW), designated GW170817, pictured left. Afterglow, designated as SSS17a, is left over from the explosion of two neutron stars that collided in galaxy NGC 4993 (shown centre). Only 10.9 hours after triggering the largest astronomical search in history, the Swope 1-m telescope at the Las Campanas Observatory in Chile discovered GW170817’s afterglow. Four days later, the image on the right shows afterglow dimming in brightness and changing from blue to red. CREDIT: Las Campanas Observatory, Carnegie Institution of Washington (Swope + Magellan) The Chandra X-ray Observatory was also watching. Chandra saw nothing at first, which was unusual. Scientists expect kilonovae to produce x-rays in jets of high-energy particles. Now scientists think there was a jet, but it wasn’t pointed at Earth. Chandra eventually detected x-rays when the jets impacted the surrounding gas and dust, causing them to widen and slow down. Then later in 2018, the x-ray emissions declined again. The x-rays have remained stable since the end of 2020. The inset x-ray image in the top picture is from Chandra data from December 2020 and January 2021. The x-rays come from both GW170917 and the host galaxy. Scientists think there could be two explanations for the steadying of x-ray emissions. On 17 August 2017, the Laser Interferometer Gravitational-Wave Observatory (LIGO) and the Virgo Interferometer detected gravitational waves from the collision between two neutron stars. Within 12 hours, observatories identified the event’s source within the lenticular galaxy NGC 4993, shown in this image gathered with the NASA/ESA Hubble Space Telescope. The associated stellar flare, a kilonova, is visible in the Hubble observations. This is the first time astronomers have observed the optical counterpart of a gravitational wave event. Hubble observed the kilonova gradually fading over six days, as shown in these observations taken between 22 and 28 August (insets). Image Credit: Hubble/NASA/ESA The first explanation is that a kind of shock is involved, akin to a sonic boom. When the cloud of debris from the kilonova slams into gas around GW170817, the material is heated. The temperature is enough to produce x-rays and can account for the steady kilonova afterglow Chandra detected. The artist’s illustration depicts this. The blue in the image is the debris responsible for the glow. The orange and red show the shock. The jets have faded over time, and the blue arcs in the image show where the jets struck surrounding material. The second explanation is entirely different. It says that the neutron star merger collapsed into a remnant black hole. In this scenario, material falling into the black hole is heated enough to emit x-rays, a known phenomenon around black holes. The team of researchers behind the new paper says that only one of the two explanations can spell out what’s happening. It would be an improbable coincidence for both sources to be producing x-rays simultaneously in the same place. They also point out that scientists have never observed a kilonova afterglow nor accretion-powered emissions like this before. Further observations should determine the cause of the x-ray afterglow. Astronomers will continue to observe GW170817 in both x-rays and radio waves. If the glow comes from the kilonova, the radio emissions should brighten in the coming months and years. But if the glow comes from material falling into a black hole, then the x-rays should stay steady or decline rapidly, but there’ll be no radio emissions over time. “Measuring the time of the peak of the kilonova afterglow, which probed the ejecta dynamics independent of shock microphysics, would offer a unique opportunity to do calorimetry of the kilonova’s fastest ejecta,” they write. This is important because it relates to whether or not the merger left a remnant black hole. If there’s a high-velocity tail in the ejecta, it can create excessive x-ray emissions that “… argues against the prompt collapse of the merger remnant into a black hole.” Astrophysicists know that black holes emit electromagnetic radiation in x-ray wavelengths. The Chandra X-ray Observatory has imaged many of them. This Chandra image shows Centaurus A, which is not part of this study but is the site of a supermassive black hole, shining brightly. Credit: X-ray: NASA/CXC/U.Birmingham/M.Burke et al. On the other hand, that same ejecta might emit “… a constant (or declining) source of X-ray emission in the next thousands of days that is not accompanied by bright radio emission.” If that’s the case, the authors say, it shows that the merger collapsed into a black hole. That represents another scientific opportunity because it “… will unveil how accretion processes work on a compact-object remnant of a BNS merger a few years after its birth.” When LIGO detected the first gravitational waves in 2016, they opened a new window into the Universe. One hundred years before their detection, Einstein predicted them in his general theory of relativity. Three researchers who played a central role in detecting GWs received the 2017 Nobel Prize in physics. Since the first detection, LIGO and Virgo have detected many more black holes and neutron star mergers. The combination of GW detections and quick and enduring follow-up electromagnetic observations have confirmed some theoretical work, including discovering that kilonovae produce heavy elements. This paper, along with other articles published on the kilonova, has confirmed theoretical predictions about these events. Astrophysicists predicted that kilonovae are a significant source of heavy elements in the Universe. The type of emissions and their flux both support that. “The spectrum and flux evolution of the kilonova emission from GW170817 was in agreement with theoretical predictions, demonstrating that mergers of neutron stars are one of the major sources of heavy elements in our Universe,” the paper says. In 2019 a team of European researchers, using data from the X-shooter instrument on ESO’s Very Large Telescope, found signatures of strontium formed in the GW170817 neutron-star merger. This artist’s impression shows two tiny but dense neutron stars when they merge and explode as a kilonova. In the foreground, we see a representation of freshly created strontium. Image Credit: ESO/L. Calçada/M. Kornmesser Scientists have learned a lot about neutron star mergers and kilonovae since the 1998 paper outlining how they work. We know they can create either a single massive neutron star or collapse into a black hole. We know the merger can create an extraordinarily powerful magnetic field that’s trillions of times more potent than Earth’s puny magnetic field and that they can make that field in milliseconds. Astrophysicists know they can produce gamma-ray bursts and that kilonovae can synthesize heavy elements like strontium. But scientists are excited about the future. “Observations of GW170817 are mapping an uncharted territory of the BNS (binary neutron star) merger phenomenology and have far-reaching theoretical implications,” the authors write in their paper. More: Press Release: The Unfolding Story of a Kilonova Told in X-raysResearch: The emergence of a new source of X-rays from the binary neutron star merger GW170817Universe Today: Hubble Has Looked at the 2017 Kilonova Explosion Almost a Dozen Times, Watching it Slowly Fade Away The post The Expanding Debris Cloud From the Kilonova Tells the Story of What Happens When Neutron Stars Collide appeared first on Universe Today.
Read original article here.
Written by: Evan Gough
Facebook Comments